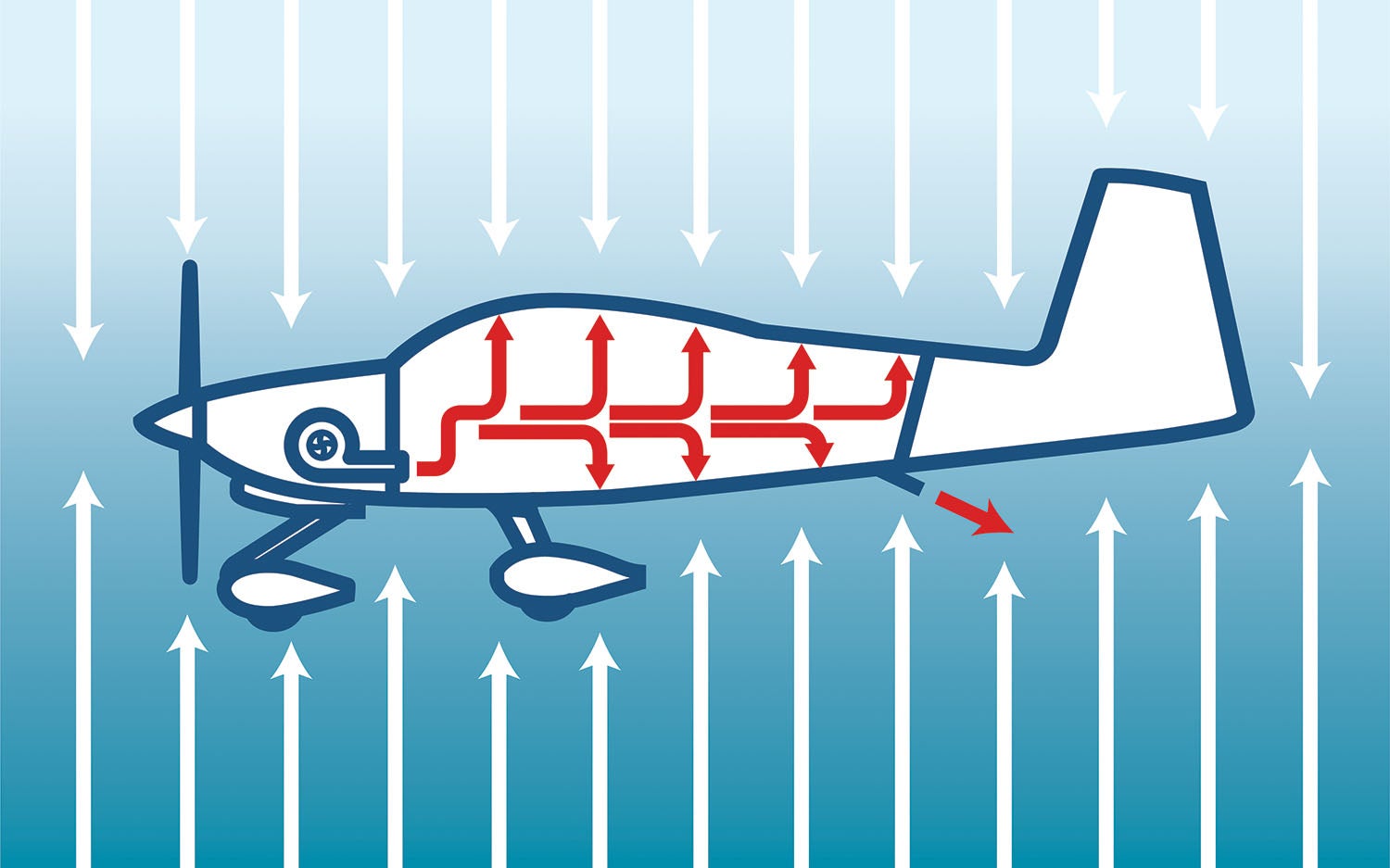
No, this working title isn’t about sitting down close to my draft submission deadline staring at a blank screen. (But it could be.) This article is about aircraft cabin pressurization, an aeronautical advancement conceived over 80 years ago that, while it has completely revolutionized airline, military, corporate and space travel, hasn’t really successfully taken off at the level of most general aviation and Experimental/Amateur-Built machines. The reasons are many—and even interesting to those of us who like to understand how things work.
Even if the technology is relatively rare in homebuilt circles, it is helpful to all of us to understand the science behind the systems. Cabin pressurization lends itself quite well to large aircraft, less well as airplanes get smaller and quite unwell to small personal-sized aircraft.
I Feel the Need
We all know that our earth’s atmosphere gets thinner with altitude. Our atmosphere is comprised of a handful of gases, including nitrogen, oxygen, argon, bovine methane and the like. To us, both as humans and as pilots, oxygen becomes the most important element because both our lungs and our jugs depend upon it to keep all of our other respective systems operating properly. Our bodies and our aircraft powerplants share a common need to breathe.
As the atmosphere thins with altitude, the amount of oxygen in a given breath decreases. Depending upon myriad factors, the decrease can bring upon a plethora of undesirable consequences to the human body, sometimes even insidiously masked by euphoria, which can make the afflicted oblivious to the dangers at hand.
Both our bodies and our aircraft propulsion systems generally do fine below about 10,000 feet. There are exceptions, but I am trying to keep things simple. In about the 1920s, mountain climbers started carrying tanks with supplemental oxygen to improve performance at high altitudes. About the same time, pilots started using very similar tanks and masks in aircraft.
Pump Me Up
Just as our bodies and our aircraft share a need for an oxygen boost at higher
altitudes, it was soon discovered that solving one problem actually might facilitate a solution to the other. For mechanical air induction systems, the solution was to compress more oxygen-dense air into the engine intakes by using turbo– or superchargers. For biologic respiratory systems, the solution was to tap off some of that compressed air and divert it to a reinforced cabin structure to pump the “cabin altitude” down to an equivalent level of a lower altitude. Win/win. (In theory.)
Experimentation with cabin pressurization began in the ‘20s, and in 1937, the Army Air Corps won a Collier trophy for the XC-35, a highly modified Lockheed Electra 10A that successfully demonstrated a high-altitude-capable aircraft with a pressurized cabin. It could maintain a cabin altitude of about 12,000 feet up to an actual altitude of 30,000. In 1938 Boeing modified its B-17 bomber into the model 307 Stratoliner, and in 1940 it was the first airliner to enter commercial service with a pressurized cabin.
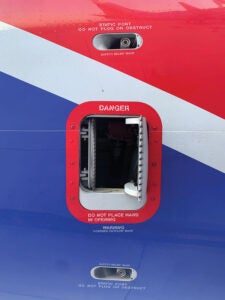
It’s Complicated
So how does pressurization function? In the simplest of terms, compressed air is directed into a reinforced cabin at greater volumes than is allowed to escape through a controlled outflow valve in order to replicate the breathing conditions of a significantly lower altitude. However, the engineering reality of both the system and the aircraft structure that contains the system is infinitely more complicated than that.
Most airliners pressurize to a differential of about 8 psi, which maintains a federally imposed limit of no higher than 8000-foot cabin altitude at cruise altitudes into the mid-40,000-feet range. Small aircraft generally pressurize to about half or less than that of transport-category aircraft. Four psi may not sound like much until you do the math. At 4 psi a 12×12-inch window has to contain nearly 600 pounds of pressure. A 24×36 door has to contain about 3500 pounds of pressure and still be able to open and close, which is why windows tend to be smaller and more rounded, and doors, especially the hinges and latches that have to carry the load, are built vault-like.
Round or oval fuselage cross-sections work best for cabin pressure containment. In contrast, boxy shapes work best for passenger comfort and head and shoulder room, so there will always be compromises either way where the less desirable effects go up as cabin size goes down. Then there’s the issue of the two pointy ends. Airliner cockpits are relatively easy to engineer for pressure containment at the front end. Still, since single-engine small aircraft engines have to have cooling airflow, the FWF area isn’t pressurized, so that means that the firewall has to be beefed up and, since it is a relatively large area, engineered to carry a massive pressure load. Empennage and tail sections on conventionally configured (non-canard) aircraft are ill-suited to pressure containment because of all the moving parts and surfaces. The solution is to employ a heavily fortified pressure bulkhead between the cabin section and tail area, often including cargo areas. This completes the pressure “vessel” but still doesn’t account for all the penetrations required for the passing of controls, cables, wires and myriad other elements in a modern aircraft. Each penetration presents a potential pressure leak point, and penetrations that involve control systems can have their own artifacts, such as a stiffening of the controls when the cabin pressurizes.
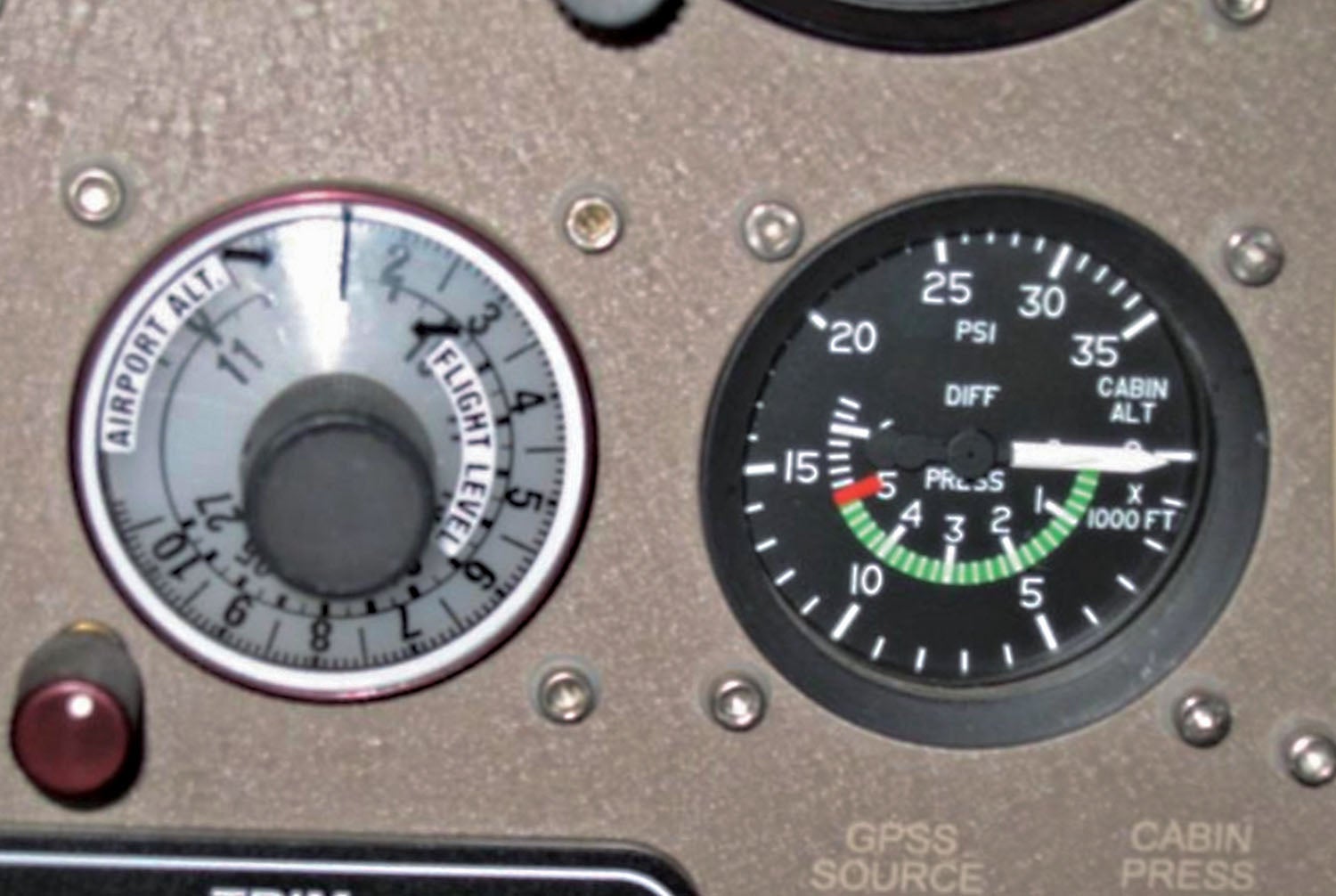
Blow Hard
The pressurization system itself is also fraught with engineering headaches. The tapping of the compressed air itself via a turbocharger or supercharger (in the case of piston aircraft) or the compressor section of a turbine powerplant inherently reduces thrust performance and fuel efficiency, which must be accounted for. Compressing air also heats it to levels too high for cabin use, so expensive and heavy intercoolers are required to pre-cool it, and complex mixer-valve chamber assemblies are often incorporated to keep the cabin air temperature comfortable at all operating regimes, sometimes even requiring supplemental Freon-type air conditioning in warmer environments. In certain situations, water vapor buildup can be a problem. Lastly, the air must be adequately filtered to prevent source contamination.
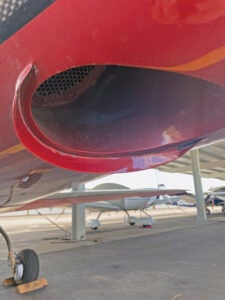
Once the source air is available and flowing into the cabin, the key to the whole process is control of the outflow valve. In simple terms, the outflow valve is the controlled leak in a pressurized system that keeps the vessel to a specified pressure differential (outside to inside). But it has to be very precisely controlled, so the occupants don’t get their ears drummed during every climb or descent. For example, an airplane might be descending at 2000-3000 fpm, but the cabin needs to be descending at no more than about 700 fpm for comfort. And it has to equalize as close to touchdown as possible because you don’t want to land with the cabin pressurized. In addition to all of the above, a proper system also needs gate protection against overpressurization and, in large airplanes, even negative pressurization. As I said, it’s complicated.
Danger, Will Robinson
There is no doubt that pressurization has revolutionized transport-category air travel. Long-range operations would not be economically feasible at breathable altitudes and unacceptable to passengers without a pressurized cabin environment. The road to success has not always been easy, however. Early pressurized models, including three DeHavilland Comets, were tragically lost due to engineering misunderstandings of window stress points in pressurized hulls. There were also issues with early Douglas DC-10s, with cargo door failures that created pressure imbalances so powerfully destructive that the main interior floors could collapse under the stress of the pressure disparity until blow-out panels were designed to allow rapid equalization.
Later issues have cropped up in older high-cycle aircraft that have had structural failures leading to rapid-depressurization events. The Aloha 737 event, where a large section of the upper cabin blew off, is one of the most infamous. Even though lives were lost, the aircraft managed to land and save most of the passengers and crew.
Another issue with pushing the envelope of the laws of physics is that in high-altitude high-pressure differential situations, a depressurization can not only instantly remove all the breathable air in the cabin but do it with such force that it literally sucks the air out of people’s lungs significantly reducing the “time of useful consciousness.” The time of cognitive recognition and presence of mind to don emergency oxygen masks gets reduced to mere seconds.
Perniciously, a slight and slow depressurization situation can sometimes be more deadly than a rapid one as pilots and passengers pass out oblivious that there is a problem until it is too late. The aircraft often continues on autopilot until it runs out of fuel and crashes, as evidenced by examples like the tragic loss of golfer Payne Stewart in a LearJet and a similar loss of a TBM aircraft en route from the upper east coast to Florida. Both were attributed to gradual pressurization loss that went undetected until it was too late. There have been several other examples. Anybody who operates a pressurized aircraft, large or small, must have extensive and recurring training on the unique operation and procedures, which is one reason why insurance companies get rather wrathful in their quotes on personal aircraft that are pressurized.
What About Us?
There certainly have been attempts and offerings for pressurized light aircraft models for both certified and Experimental markets. Beechcraft offered models of its B58 Baron in pressurized form, and Cessna did the same for its C210 Centurion line, but both offerings were only sold for a comparatively small production run compared to their un-inflated stablemates. Mooney managed to produce only 36 copies of its M22 pressurized model, which had to be sold significantly below cost. Piper says its M350 Malibu is the only new pressurized piston single on the market today.
On the E/A-B front, the Lancair IV-P and its big brother spin-off Evolution series aircraft kits were pressurized, but kits for neither are currently offered new for sale. Both models have recorded incidents of depressurization issues involving separated windows and doors, and in the case of a couple of Evos, separated windshields. The controversial Raptor project was to be pressurized, but its designer has decided to scrap that design; the Raptor NG will not be pressurized due to the “weight and complexity” of the feature. I’m sure there are some Beech or Cessna engineers saying, “I could have told you so.”
No doubt some other entity will eventually attempt to make another pressurized offering to kit builders. I remain severely skeptical but will gladly listen. The economic headwind is really two-fold. First, pressurization tech scales poorly downward, taking a disproportionately large chunk of time, money and payload with it. Second, for most pilots in this arena, the desire to fly higher (for terrain, weather, tailwinds, ride comfort) can be met with simple and relatively inexpensive supplemental oxygen systems. Suppose a primary goal is the luxury of not having to use supplemental oxygen. In that case, the fact remains that to legally and safely use altitudes above breathing levels, supplemental oxygen for each occupant must still be carried and available even if the aircraft is pressurized, minimizing the benefit and augmenting the associated weight, complexity and cost penalty.
No doubt Lancair IV-P owners will respond that their system is all that and a bag of chips. It may be, but there is a difference between technological feasibility and realistic market acceptability, and if those systems met the latter, they would still be available. They aren’t, even in the new Lancair incarnations. (Although rumored to possibly be considered in the future.)
Aviation technology has changed over the last several decades, but the laws of physics have not. As Occam’s Razor avers, sometimes the simplest solution is the best. Even with 90% of my logged flight time in pressurized aircraft, my occasional need for high-altitude operation in my personal aircraft is adequately met with a simple and cost-effective portable oxygen bottle and nasal cannulas in a strong but light and cost-efficient hull with nice sized windows. The general aviation market, so far at least, seems to agree.
Illustration: Peter Jones; Photos: Myron Nelson.
I would have to disagree with Myron when he asserts that the economics drive the no-pressurize decision. I would say it’s closer to laziness. It’s obviously been done, and it follows that it could be done better. What if we said it’s too hard to pack so many pixels on a retina screen? or it’s too hard to use a lithium battery instead of alkaline? or it’s too hard to build a precision approach out of GPS.
Somehow aviation lives in this nether world where so many things are just “too hard”.
I have to disagree with you about things being to hard. Nothing is to hard if it is profitable. So I believe the demand for single engine pressurized aircraft is to small for it to be profitable. I might stretch that small twins 6 place and smaller. In my lifetime nothing that is profitable and not new technology is never to hard. Money motivates. Cool and neat goes broke. Unless of course there is money.
I have a Lancair 4 and it is not pressurized. I assure you that it is not due to laziness. With a useful load in the neighborhood of 1000lb dedicating 125lb to the comfort of pressurization substantially reduces range.