Editor’s Note: It’s not common we get to test similar products in closely controlled conditions, and it’s also not common that we get to test similar products from basically dissimilar companies. Here we have two modern three-blade propellers that Editor at Large Paul Dye was able to test on his RV-8 over the course of a few months, with one prop coming from industry stalwart Hartzell and the other from Whirl Wind Propellers, a company well known in the Experimental world. Whirl Wind’s Jim Rust and his company have been working for decades refining composite-blade props for Experimental aircraft (among other applications).
Paul is open to trying a lot of things, and our benefit is that he has lots of time on his RV-8, and extensive data from it using a more conventional aluminum blended-airfoil (BA) Hartzell two-blade prop. Another benefit is that his RV-8 is powered by a standard-issue O-360 Lycoming clone, meaning the comparative data we get here could stand in for a lot of engine/airframe combinations.
Last year, Whirl Wind asked if Paul would like to test the latest three-blade, and we published the results in the January issue. It offered the expected improvements in takeoff and climb, but failed to render the usual reduction in cruise speed you see when adding a third blade.Hartzell, for its part, has been developing new composite-blade props into the certified market and has created several iterations, from bush-oriented low-speed props to those intended for faster aircraft. The new Explorer prop was just out when Hartzell asked if Paul would like to test it as well. So what was never really intended to be a prop “shootout” ended up being a very interesting look at prop-performance data. I’ll let Paul pick up the story from here.
—Marc Cook
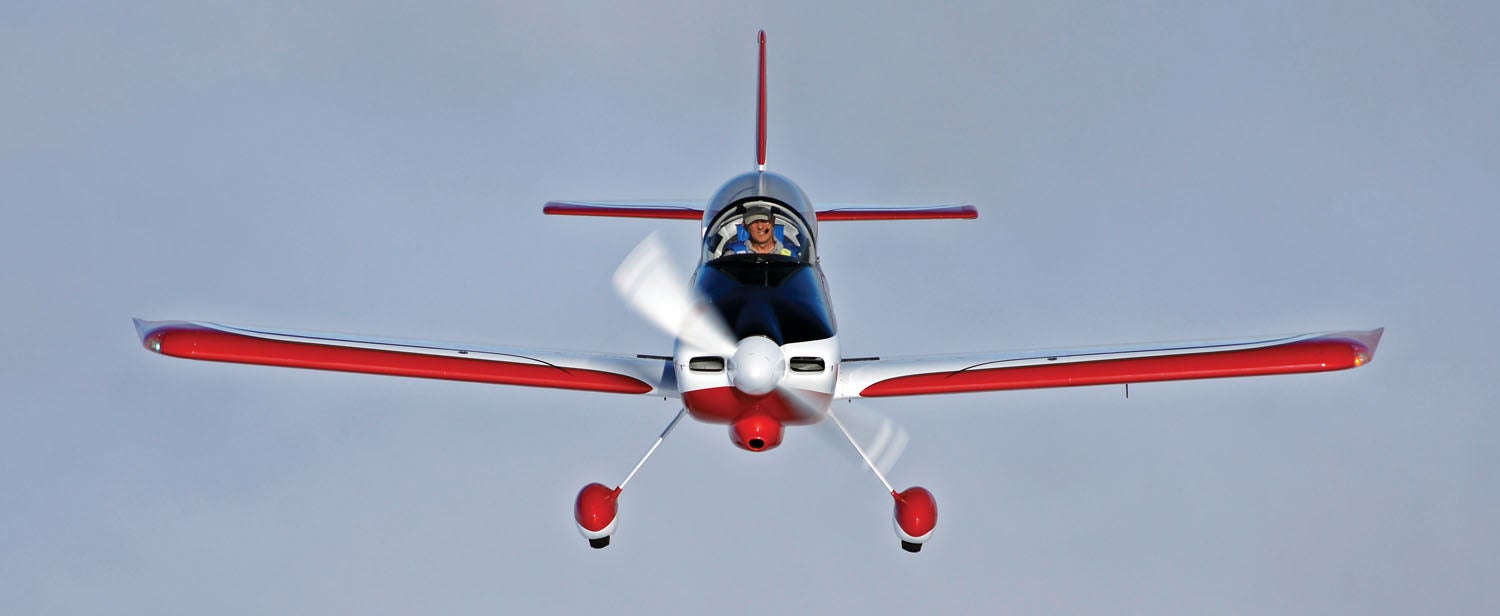
So What’s New? A Little History…
Early on in Experimental/Amateur-Built aircraft, they gained some power and performance and then quickly outgrew the fixed-pitch props popular at the time. Soon the engines and props from damaged Piper Arrows and Mooneys began appearing on the noses of new Experimentals at Oshkosh. That meant constant-speed props, usually McCauley or Hartzell. The standard Hartzell two-blade prop—the one some of us refer to as the “paddle blade”—reigned for years until Hartzell recognized the sheer size of the RV fleet and came out with the so-called blended-airfoil (BA) design in metal.
I remember a noted member of the RV community who did a head-to-head comparison between the older Hartzell and a new BA prop on the same airplane, and described a 5-knot speed gain. At the time, the price difference between the two props was about $400, and he described those 5 knots as “the cheapest five knots you will ever find!” This prop became a mainstay, especially among RV builders.
Now we’re in the world of composite-blade propellers, of which Hartzell was an early entrant—the Porsche-powered Mooney PFM had one in the late 1980s. And, of course, MT had been around with ’glass-covered wood-core props before that. Whirlwind Propellers began in 1995 building true composite blades for fixed-pitch and ground-adjustable applications. Whirl Wind Aviation, in case you’re confused, is the company selling versions of those blades into constant-speed hubs. Now you’re up to date.
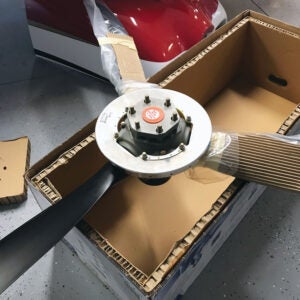
The Tests
The baseline is the Hartzell BA prop on the RV-8. More specifically, the compact hub fitted with 7497 blades. The installed weight of the prop is 58 pounds with a standard Van’s fiberglass spinner.
For Hartzell’s latest three-blade effort, there’s the Explorer. In this case, the prop has a Raptor hub and 76CS03-2X2 blades. According to Hartzell, the hub has a trade name of Raptor and can be fitted with different blades for different purposes. I asked Trevor Parker, their engineer, if the Explorer is a “speed prop” for planes like RVs or if it was targeted at the STOL crowd. He said it is designed as a speed prop, and that they have other blade designs for bushplanes. The Explorer has a broader chord than the Whirl Wind 300 and looks to me to be more of a “puller” than a go-fast prop. (We’ll see what the numbers say.) The installed weight of the Hartzell prop and spinner is 50 pounds, but that is with steel ferrules. Hartzell requires these for a non-counterweighted crankshaft, as we have on the O-360-A1A. For a counterweighted-crankshaft engine, where the power pulses are smoothed out, there’s a lighter aluminum ferrule that lowers the all-up weight by 8 pounds.
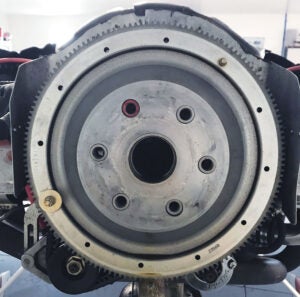
From Whirl Wind is the WW-300. Jim Rust’s latest three-blade prop has his own hub design and pretty skinny blades compared to the Hartzell. We wrote up our test results in detail in the January 2021 issue of KITPLANES®. This is the lightest of the three props we tested, coming in at just 34 pounds, installed. Jim Rust is happy with the configuration of the blades on the non-counterweighted crankshaft. Another bonus: At least for an RV-8, which is always fighting a tendency toward nose-heaviness with a constant-speed prop, lighter is definitely better.
The Test Plan
For each propeller, we wanted to find three simple things: how fast it would go at various engine rpm and cruise altitudes, how well it climbed, and how quickly it got you off the ground. Traditionally, two-blade props are faster than three-blade props of the same design, while three-blade props pull better, giving better climb and shorter takeoff rolls. In our case, we expected to see some interesting results because it wasn’t just a matter of blade counts: We were also comparing new blade designs to older shapes. We weren’t expecting to see huge performance differences, so getting very accurate data is crucially important.
The standard flight plan was to fill the fuel tanks and ballast the airplane to the same CG for each prop (to eliminate speed differences due to trim drag), then line up on the forward edge of the runway threshold line. We had an assistant down the runway at the expected takeoff point to note where the mains left the ground. Each takeoff started with a full-power run-up with brakes held, then a release at maximum rpm with the stick forward. As the tail came up, we caught the pitch at level and let the airplane fly off. You could probably get a slightly shorter takeoff than with this technique, but this was consistent from prop to prop, and that’s what matters.
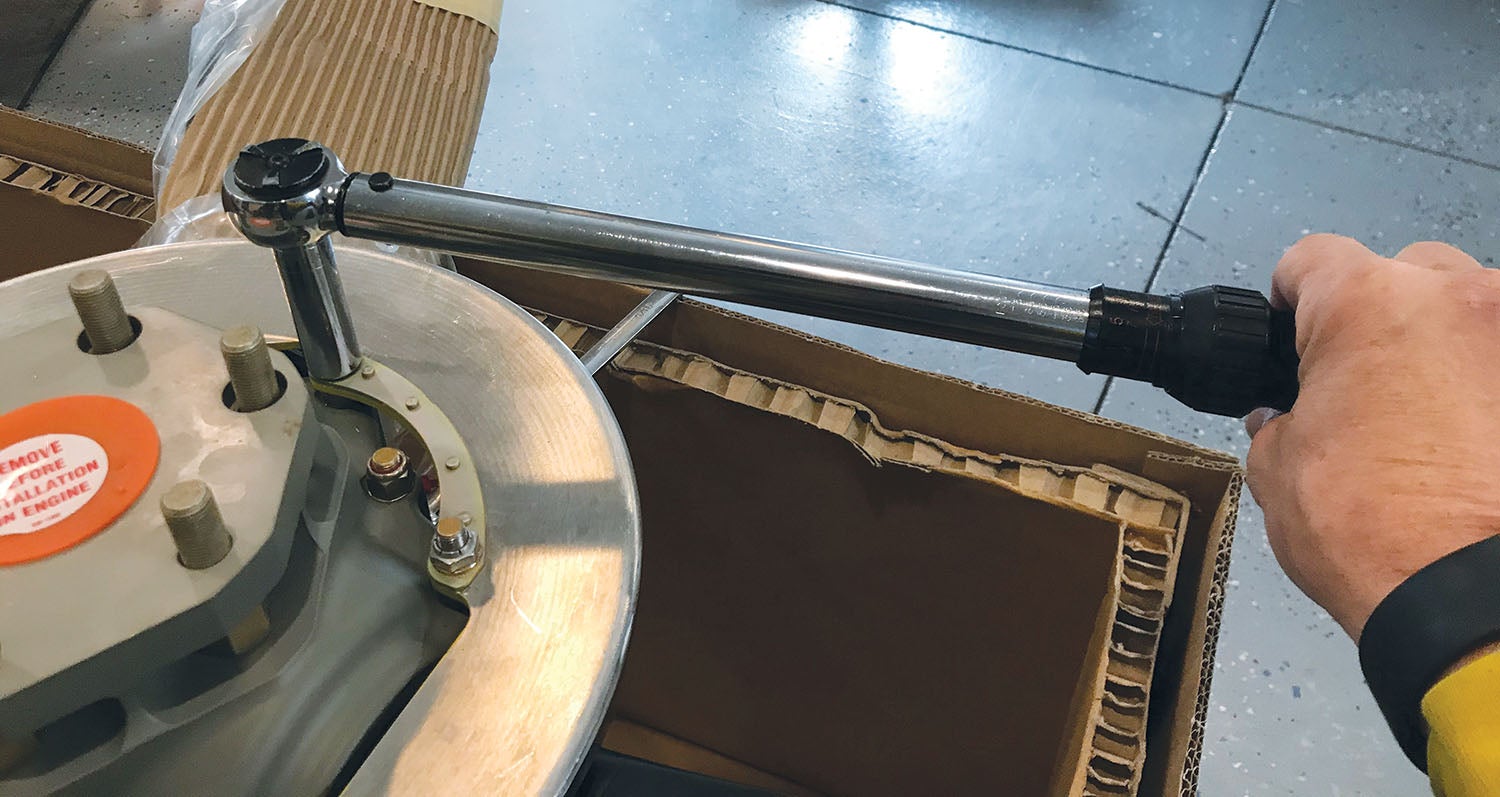
As our ground observer marked the takeoff point, we climbed out on a repeatable flight path (so we burned about the same amount of fuel) to the start of the speed test run at a density altitude of 8000 feet, as determined by our GRT EFIS. Upon reaching altitude, we let the TruTrak autopilot hold altitude while we tested for maximum speed at each rpm setting on the card. This involved going to maximum rpm, then leaning to best power via EGTs and fuel flow. After letting the speed settle out, we recorded IAS and TAS as well as the computed percent power from the EFIS. Then we reduced engine speed to 2600 rpm and repeated the leaning and settling, then dropped it to 2500, 2400 and 2300 rpm, carefully letting things settle out each time.
By this point, we’d covered about 30 miles in a straight line, so we were out over the desert test area. Next up was climb testing. Before beginning the prop comparison tests, we had gone out and repeated our Phase I testing (to confirm that nothing had changed and to refine the piloting task) to figure out that the best rate of climb in the airframe was between 95 and 100 knots indicated at 8000 feet. This allowed us to quickly do just two tests for each prop instead of an entire sweep of the speed ranges.
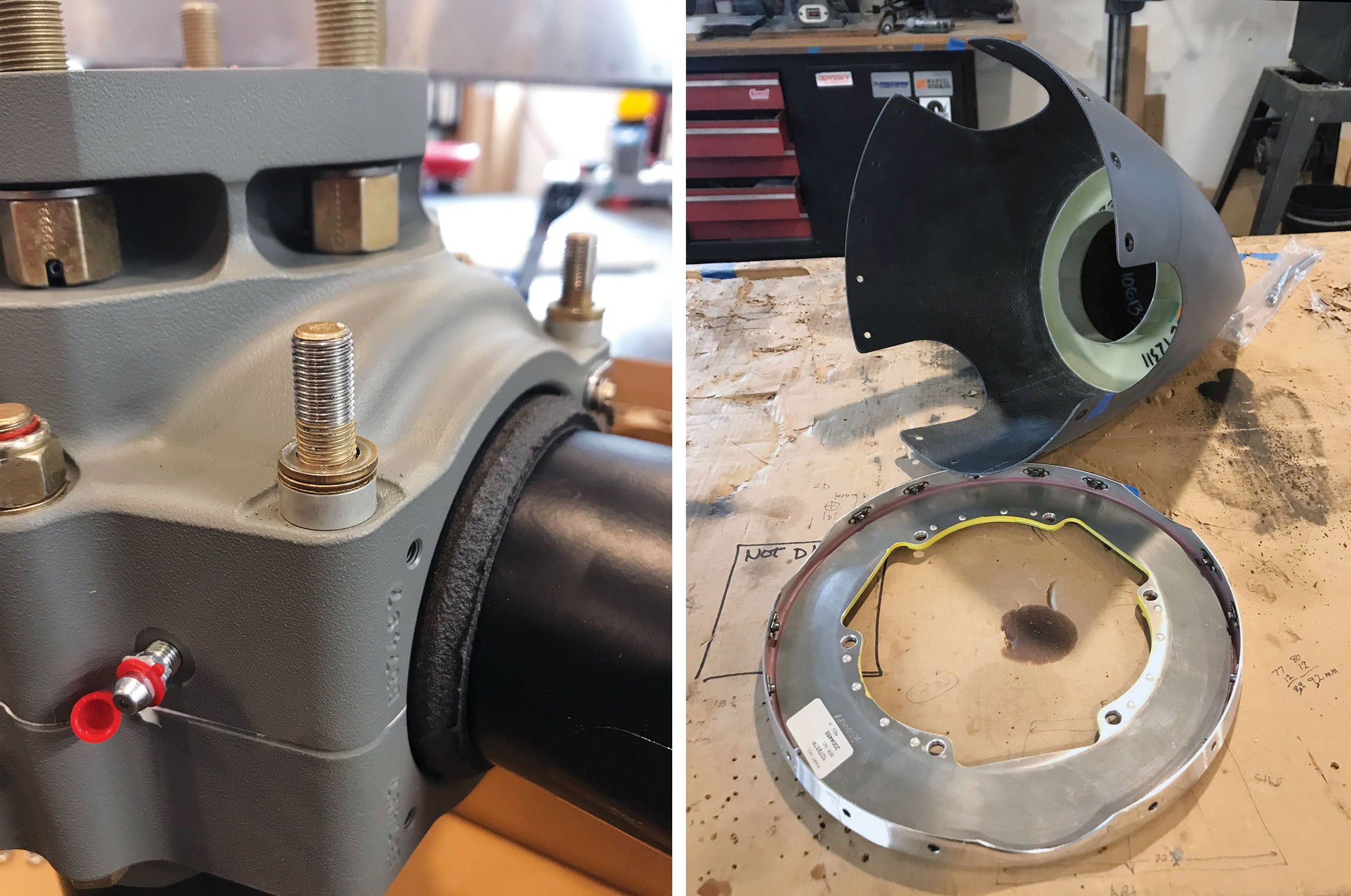
For each climb, we started by diving the airplane to 7000 feet DA with the throttle at idle, then leveling off and slowing to the speed at which we wanted to climb. We then pitched up to capture that speed and added power to begin the climb. Start the stopwatch passing through 8000 feet DA and stop it passing through 9000 feet DA. We ran the test twice for climb speeds of 95 KIAS and 100 KIAS.
The last test in the flight was to climb to 14,000 feet DA for a repeat of the speed runs to see how blade performance varied with attitude. The climb was done at best power to maintain common fuel flows, and once we leveled off, the exact same speed test was flown as we did at 8000 feet DA.
One of the things we had to watch out for when letting the autopilot set pitch was atmospheric waves caused by the mountains to the west. This is why each test was performed first thing in the morning on calm days. A wave in the atmosphere will cause you to fly in and out of alternately rising and sinking air and, if the autopilot is trying to hold attitude, that will cause the airplane to pitch up (slow down) and pitch down (speed up) while maintaining a constant attitude. Sometimes these artifacts are subtle enough that they’re difficult to notice, but they will throw off the data.
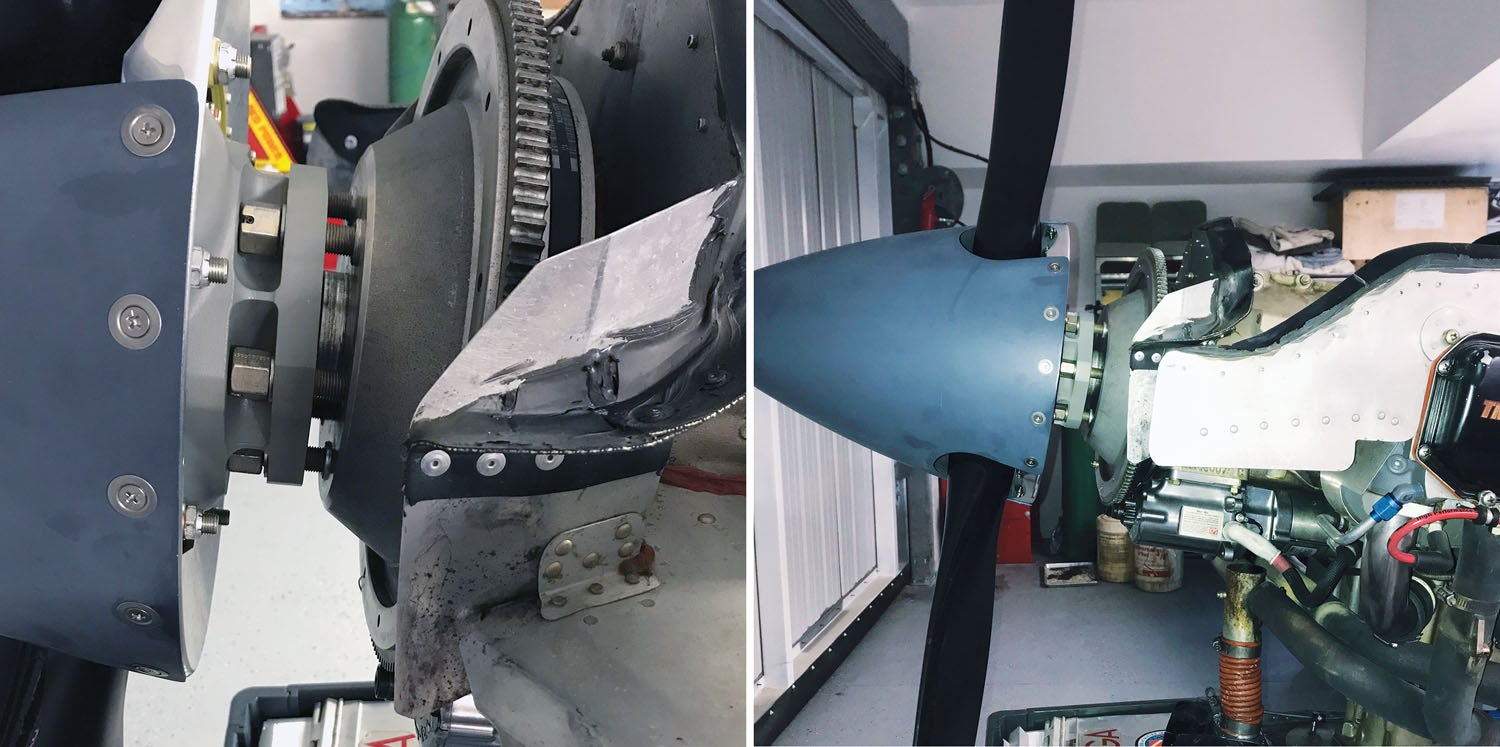
This characteristic of flying near the mountains requires careful observation to make sure the data isn’t being polluted—even with calm morning winds, the leftover waves from the previous day and night can upset the careful collection of data. So we tried to get far enough away from the mountains for it not to be a factor. And even then we had to repeat the data flights several times. Proper mixture leaning can have dramatic results on the power you’re getting out of the engine, and while EGTs are a good first approximation and the power numbers generated by the EFIS are good indications, fuel flow was the final determinant of getting repeatable data. This is a critical consideration when you’re making direct comparisons.
Finally, Data!
According to Trevor Parker, Hartzell propeller engineer, “It’s really hard to design a really bad prop. It is also really hard to design a really good prop!” And our testing proved that. All three props for which we collected data do a good job of turning horsepower into thrust—the more modern designs doing a slightly better job than the older design, but the tried and true still satisfies. We also saw some interesting, albeit unexpected results that, in retrospect, make some aerodynamic sense.
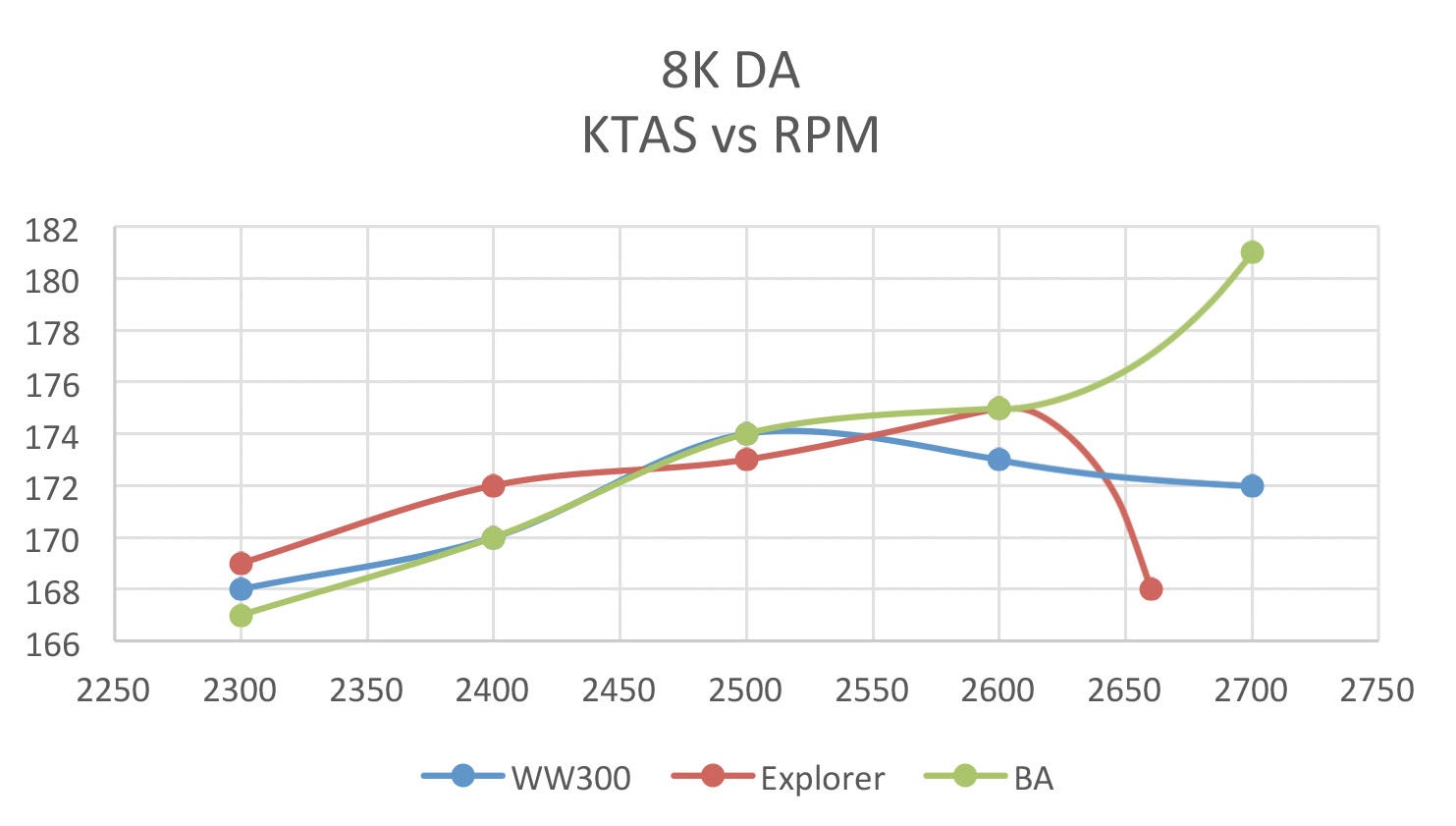
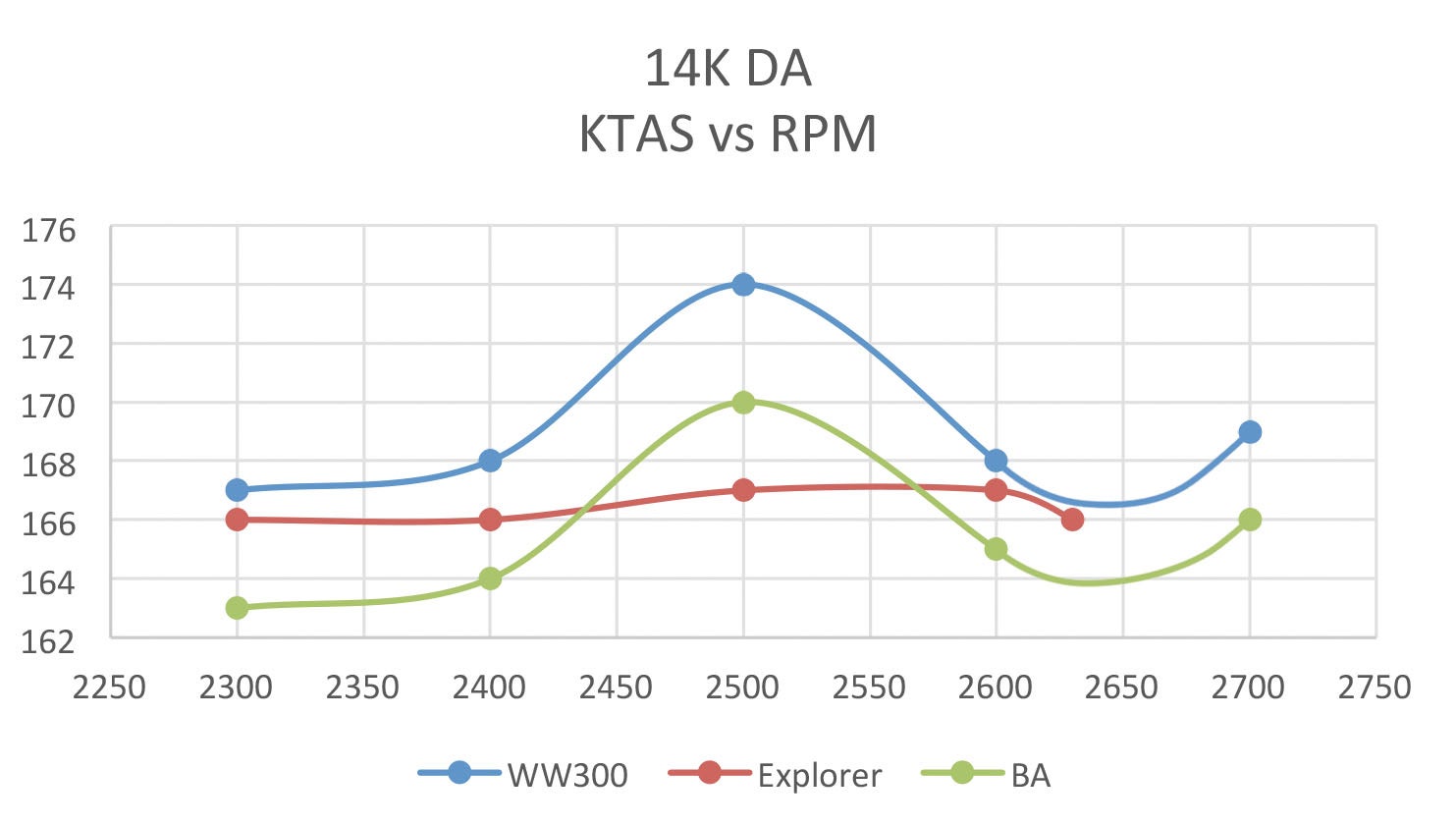
What we see in this data is that the two-blade BA (7497 blades) at 8000 feet DA just keeps going faster as you increase rpm to redline. Both three-blade props tend to drop off at maximum rpm but have a fairly broad range of maximum speed, over like a 200-rpm range. At higher altitude, in this case 14,000 feet DA, the Explorer behaves very much the same as it does at 8000 feet. But both the two-blade BA and the WW-300 have a very distinct speed peak at 2500 rpm. And the Whirl Wind is actually the fastest of all of them at that altitude.
So, if you want to go the fastest around the pylons at Reno in September, you probably want the two-blade BA prop. If you want to go fastest at altitude, you want the WW-300. If you want to climb and take off better, you want either of the three-blades—they are virtually in a dead heat for takeoff and climb performance. If you want a lighter nose, you want the Whirl Wind first, the Explorer second and the metal two-blade third. An RV-6 is naturally tail heavy, so the Explorer might be a better choice if you’re shopping for a three-blade prop.
Of course, all this testing is on an RV, which does not answer the question of how these props would work on a slower, STOL-biased aircraft. Manufacturers target specific designs for specific jobs, and Hartzell, for instance, has a different prop optimized for the “off road” crowd. Since we have a Dream Tundra sitting in our hangar with a two-blade metal Hartzell BA prop on the nose, we’ll have to go through the test card yet again, then hang the Explorer on the nose to see the differences. That will have to be for a future article—and maybe we’ll find yet another prop to try at the same time.
As Parker’s quote suggests, the differences between well-designed props that are targeted for a specific task are generally going to be small—flight testing for those differences requires patience, precision and the willingness to throw away a flight’s worth of data if it is later determined that the atmosphere wasn’t right to give consistent results. Like testing for minor drag reduction efforts on an airframe, you have to be smooth and repeatable—and that makes getting good quality data all the more fun and rewarding. It’s a great excuse to make yourself a better pilot!
Photos: Paul Dye and Kai Hansen (courtesy of Paul Dye).
Regarding the prop comparison, it is stated that to simplify the testing the best climb speed testing from phase 1 was repeated. It was implied that this was a test of the airframe. In fact it is not. Rate of climb is equal to excess thrust/weight, so clearly the engine and prop are implicated. You change the prop and your best climb speed could change. Also, why plot speed vs rpm? What is that telling you about efficiency? I would have preferred speed vs BHP. Finally, I question the oft repeated claim that 3 blade props climb better and 2 blade props go faster, when considering blades of the “same design”. The blade design to absorb X hp with 2 vs 3 blades will be quite different. It is a very difficult claim to prove, since prop performance is dependent on how well the prop is matched to the engine and the airframe. It is difficult to separate what is due to blade count and what is due to being well matched to the engine/airframe combo. I think this is another of many airport coffeeshop myths.
Thanks for reading the article, and for your comments. As you know, flight testing and engineering is often about specific choices about the data you want to collect and which parameters you want to control. In these tests we chose to use RPM as our comparison point for speed because it was easily measured with no other data reduction required. Yes we could have compared speed to BHP, and since we recorded Percent horsepower for each data point that could be backed out if we wanted to do so. But we were mostly interested in the variation of speed with prop RPM because it was looking (in a roundabout way) at airspeed past the prop. It was simply a choice. AS to the best rate of climb speed the truth is that the actual best rate of climb changes very little over a broad speed range for an RV – finding the actual Vy is tricky to the point of distraction because a very tiny change is buried in the noise and uncertainty of the test. The different props were not radically different enough to significantly change the best rate so we picked arbitrary but repeatable -speeds.
Remember that this was about comparing props at specific conditions, not determining the absolute performance of a specific prop. Doing a comparison is actually easier and relatively quicker than doing a complete parameterization of any one design.
Did I miss something – I dont see the data on the climb performance.
Craig, you’re absolutely right! I guess that once we determined that the three-blade props were virtually identical in climb performance (we did mention that in the text, but didn’t highlight it), we sort of forgot to write anything more about it, our include the data. In summary, the three blades were as close as we could measure in climb rate, and they were both about 10% better than the two blade.
Did you notice any significant difference in ride quality, vibration or noise between the various propellers?
Roland – no, all three props were very smooth, and give a powerful feel in a solid airplane. Of course, we did dynamically balance all the installations before flying them, so we expected things to be smooth.
Are you familiar with Hartzell’s “D twist” blade? From what I’ve been able to gather it’s very similar to what Dave Anders had his Hartzell twisted to for his very clean and fast RV-4. For years I looked for a prop shop to twist my blades. Then I stumbled on an STC for the Twin Comanche
https://www.knots2u.net/speed-propellers-set-of-two-piper-twin-comanche-pa-30-pa-39/
The speed increase on Dave’s RV-4 was significant! But as far as I know, no one has tested the blades on a stock RV. The “D twist” blades may be the best kept speed secret! Or not…
For the average RV guy it would be an expensive gamble to try.
Hello Dave,
I recall hearing of the “D twist”, but have never looked into in detail. My guess (purely a gues) is that the technology and aerodynamics of composite props has overtaken them. In other words, any proven gains from the profile have undoubtedly been incorporated into the designs of the composite blades – no designer leaves performance on the table if they have a choice! On the other hand, we have to remember that “performance” is a slippery word – if you increase climb performance, you generally lose som speed…and vice versa. So in picking props, you have to decide where you want your “sweet spot” – many go for overall speed, while others (particularly those in the mountains) will prioritize climb.
You’re right that it would be an expensive test for an individual if they couldn’t return the prop if it didn’t measure up!