Lee Riedel perches in the copilot’s seat and squints at the screen of his laptop computer resting, well, in his lap. “OK,” he says, “we need another one. Let’s try it with 15 inches of manifold pressure.” As we complete the climb back to 10,000 feet, Riedel pores over the data one more time, looking for demons hiding among the squiggly lines. A steady stream of data is humming back from a special carbon-fiber enclosure on the prop hub that looks like a tiny black hat. At the top of the climb, he indicates that he’s ready. So without much in the way of concern for the engine, I pull the throttle back to 15 inches and run the prop control back to the max setting. A quick glance across the cockpit to make sure Lee is ready…and over goes the nose. California desert fills the windshield as the Sportsman picks up speed. An initial pitch-over of 30° seems to work well. Another quick glance to the right, looking for 2750 rpm on the tach, and it’s evident we’re almost there. Just a little more pitch angle…
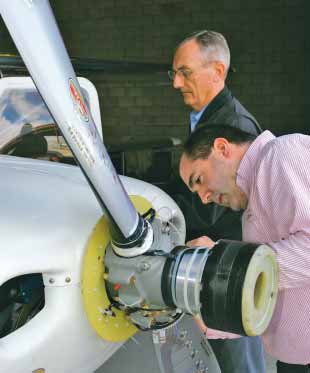
“Good, start the pitch-up,” Riedel calls. I gently pull the Sportsman toward level flight, aware of the rush of wind noise and the airspeed needle edging around the glass to the redline. I continue the pitch-up maneuver as the horizon slides down the frame members in the windshield and then slithers below the cowling. It’s now just bright sunshine and high-altitude clouds in my view. It’s quiet at last. The engine gives a mild shudder coming through 70 knots, nose high. Eventually, as the airplane sacrifices airspeed, the pitch forces lighten, the stick is moving back toward my stomach with that familiar sloppy glide that always occurs near the stall. There’s a gentle burble at the stick and a slight pitch down. A tiny bit of rudder keeps the ball in the center. I’ve hit the aft pitch stop and that’s all there is.
“That looked good,” Riedel says. “Now, let’s try one more at, oh, 17 inches and see how it does.” Great. Here we go again…
More Than Fun Maneuvers
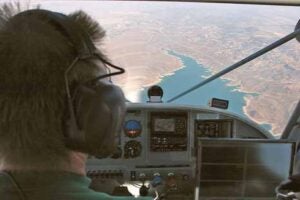
Quite a ride, isn’t it? What you’ve seen described is not some newfangled stall program or a basic introduction to aerobatics with a laptop, but just one element of an intense and exacting science involving the measurement of propeller vibration. In truth, what has come to be known as a prop vibration survey is more accurately described as a search for dangerous, metal-eating harmonics. All props and engines face distinct challenges in getting along. Certain engines create certain kinds of vibration, from big pulses down in the 1P range (sometimes called “one per,” or one beat per crankshaft revolution) as well as fractional thumps. The terminology can be confusing, it’s true, but any excitation that happens once per crankshaft revolution is colloquially known as a 1P but more accurately called a first-order vibration. A pulse that occurs twice for every revolution of the crank is a second-order vibration, and so on. The lower the frequency, the more this vibration will feel like a rumble. But there are also harmonics that go well up the range, albeit at lower amplitudes than the 1P boomers we all generally tend to think of as “vibration.”
These high-frequency oscillations are the ones that hurt metal propellers the most. In fact, when you’re flying along with what feels like a nasty propeller shake—it’s probably in the 1P or 2P (first or second order) region—the prop is actually comparatively happy.
No, the trouble starts when the prop and engine combination exhibit resonances—where a vibration created by the engine sets up a sympathetic vibration in the propeller. Think about this: The engine is creating all sorts of vibration types, low and high frequency, regular and irregular. You have natural imbalances in rotational parts, inherent imbalances in reciprocating parts, and then you have variations in the size, duration and timing of each power pulse. Change the power setting or mixture strength, and you’ve changed the vibration structure as well.
Obviously, finding that sweet spot is a moving target. The goal is to get the engine’s and prop’s natural frequencies far enough apart that they never coincide during normal operation. Doing so may require modifications to the prop, either its static balance, mass distribution, or, more commonly, by selecting a thicker blade that not only has a different natural frequency than a thinner one, but is capable of taking more of a pounding.
Why the Bother?
Because metal fatigues, that’s why, and the fatigue life is directly related to cycles. Hartzell’s literature offers an interesting observation. If the point of high stress is at 10 or 12P (cycles per crankshaft revolution), the total number of stress cycles can add up rapidly. At 10P and 2500 rpm, it works out to 1 billion cycles in 667 hours of flying. Cyclic stress at 1P for the same amount of time is, obviously, one tenth of that, theoretically prolonging the life of the blade and hub by a like amount.
Now, the big disclaimer, and one that I heard in many small stories during this week of testing: There are just too many variables to be able to predict when a prop will fail or a hub will crack given certain exposure to vibration. (Remember that not all of the vibration comes from sources that are consistent within the engine. There are power pulses to contend with as well as aerodynamic effects.) Hartzell has amassed a tremendous amount of experience with metal-blade props and has set understandably conservative limits on the frequency and amplitude of vibration certain types of props are intended to endure.
A Match Made in Piqua
In the certified world, engines and propellers are tested as mated pairs as a matter of course. It’s usually a fine dance between the airframe manufacturer, who invariably wants the lightest, fastest, cheapest prop on the shelf, and the propeller manufacturer, who wants to provide a prop/hub combination that’ll have a good service life—and by extension customer satisfaction. Sometimes the two parties have an easy time of it, all goes smoothly, and the customer gets the best of all worlds. Sometimes the engine/prop/airframe combination just doesn’t want to play. One good example is the Mooney 201, which has a couple of yellow arcs on the tach prohibiting continuous operation there. These arcs are the result of testing that showed high resonances that would build up stress cycles rapidly.
Experimental aircraft don’t always have the luxury of this testing, and many manufacturers take the tactic of choosing engine/prop combinations that have been approved elsewhere. This is certain.ly better than selecting carelessly from the brochures, but it’s not everything. In fact, there can be airframe interactions that can either improve or degrade the vibration margins, and there are modifications that many builders try that can make similar changes. For example, electronic ignition can change the character of the power pulse to a degree that it might alter the vibration signature. Enough to set up early fatigue? You’ll never know without testing.
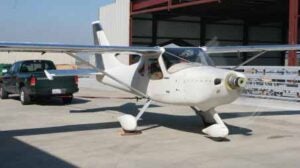
Testing, On My Doorstep
Three parties wanted to know for sure if the specific combination of the Hartzell blended-airfoil two-blade prop (on the compact hub), Lycoming IO-390 and Sportsman 2+2 airframe was a happy marriage. They were, no surprise, Glasair Aviation, Lycoming and Hartzell, which promptly dispatched test engineers John Hartmann and Lee Riedel. As I was the first to fly with this particular combination, it was my good fortune to offer N30KP for the tests.
John and Lee arrived on a Sunday night ready for work first thing Monday morning. They had sent ahead enough equipment to launch a rocket, including two prepared propellers. One was the same blended-airfoil prop I’d already been flying for 40 hours (at the time of the test), and the other was the 80-inch “seaplane” prop already used successfully with the O-360 on other GlaStars. (We did not test the 7666 prop, which is a popular combination with the 200-horsepower IO-360.)
These props had been treated with dozens of film strain gauges, about a quarter-inch long and an eighth-inch wide. Typically, they are bonded onto various surfaces and buried in the shank of the blade as well as the hub. Hairlike wires extend toward the hub under a thin coat of epoxy. Their placement is the result of thousands of hours of test data as well as computer modeling showing where the highest stresses are likely to be found.
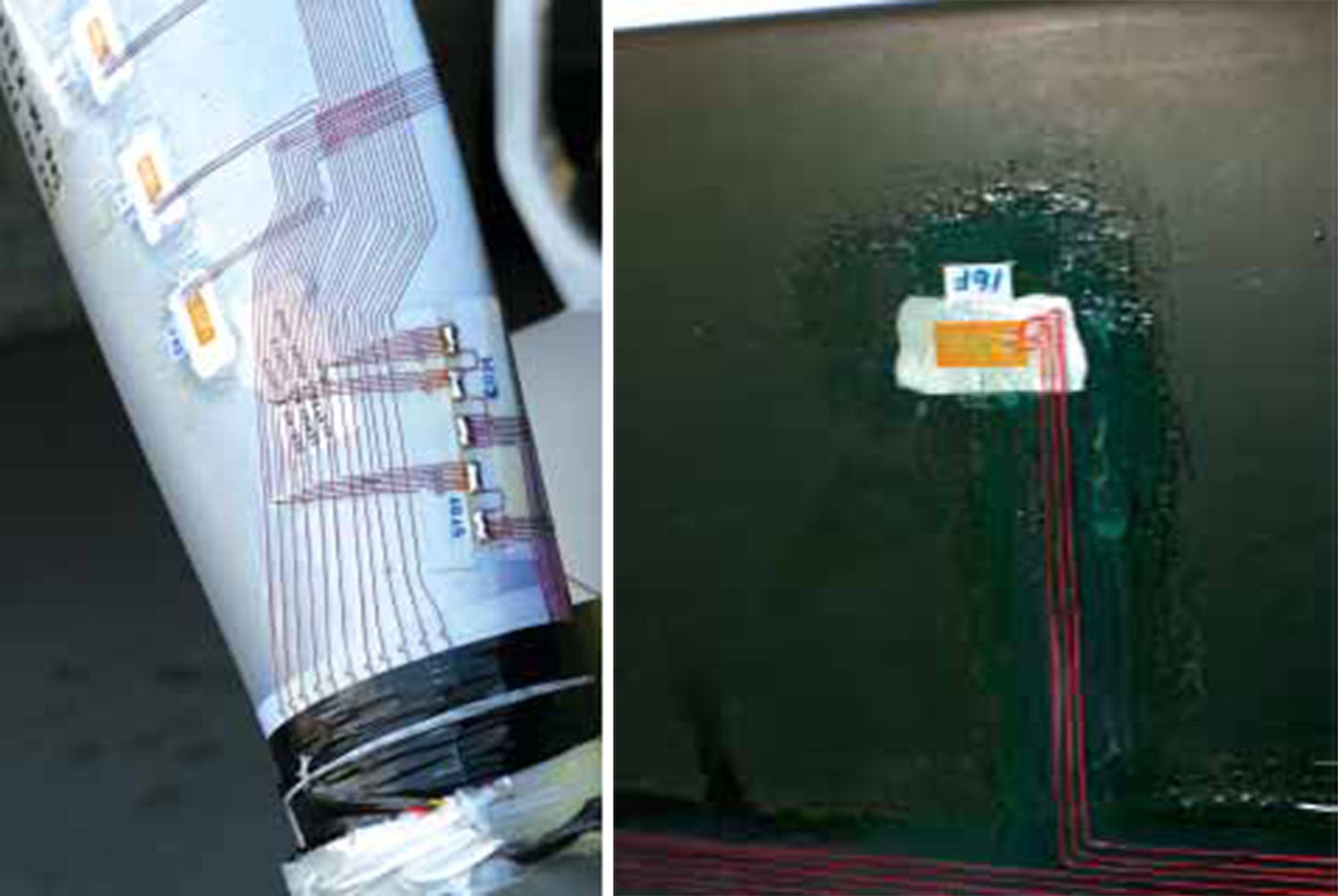
Network Activities
Signals from the tiny strain gauges are collected at special doughnut-shaped component surrounding the normal prop dome, ahead of the blades. Inside are parallel analog-to-digital converters, a matrixer and a simple RF transmitter. It’s all battery powered and doesn’t even have to touch the airframe. (This system is, I’m told, a massive improvement over transmitting by slip rings, which were prone to wear and had a limited number of channels.) This system can send 16 channels of data simultaneously but, typical of engineers, they want to measure more than that. So some tests are performed twice to rule out high vibration from areas where it’s not expected; the bulk of the testing is done with the sensors active in the worst places, notably the mid span of the blade.
On the cowling side of the prop is a simple wire antenna and a pickup that proves a once-per-revolution count for the system. We ran the wires back along the cowling under aluminum tape and into the cabin through a ventilation duct. Although the team uses a laptop to gather and examine the data, a parallel stream is sent to a 60-pound data collector with a tape drive. I looks like a first-generation VCR and probably costs as much as my engine. It took John and Lee most of a day to prep the airplane and dress the props; good thing, it rained all that day.
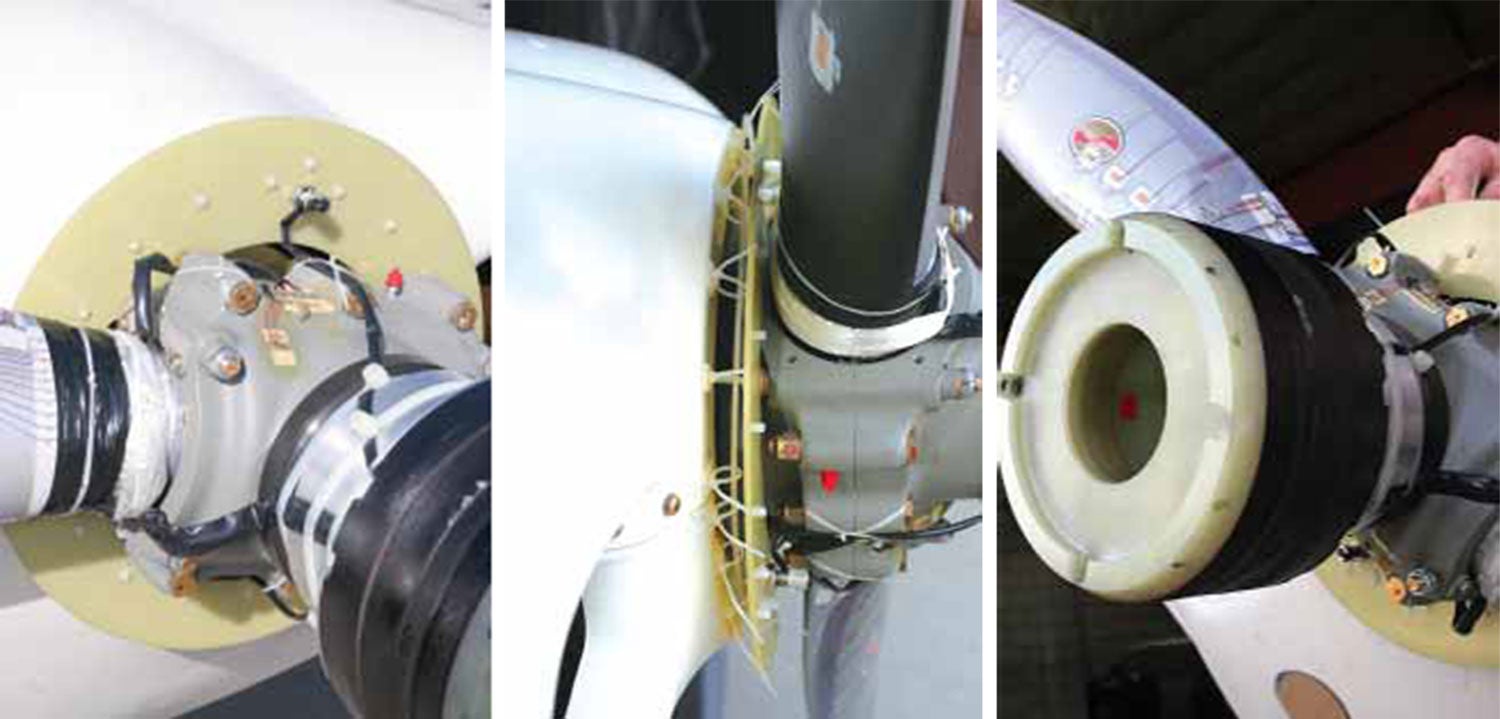
Let the Punishment Begin
The first test is done on the ground, preferably with the airplane facing into the wind. We tied the airplane to my pickup truck so it wouldn’t careen down the ramp and, with the data recorders going, it’s a simple matter of running the engine from idle to redline-plus-50 in 50-rpm increments. OK, maybe it’s not that simple. This engine doesn’t like to idle smoothly above 900 rpm but below about 1200, so hitting the 50-rpm increments precisely takes some trial and error with the throttle.
But that test isn’t as nerve jangling as the full-throttle test, where you start with max power (again at redline plus 50 rpm) and work down to 2000 rpm using the prop governor. This would be the most stressful part of the testing—for me. We managed to keep the peak CHTs to around 420°, a number that had until that time never been reached, and has not been seen since. With the torturous ground tests done, we began the more exciting part: flying. Hartzell’s test program consists of two primary flight tests, with a handful of smaller tests, to check for harmful vibration. To examine for the influence of the power pulse, one test is committed at as low a level as is safe, thereby obtaining maximum power. This one, I have to say, was a hoot, scampering along at approximately 2000 feet msl with the throttle pinned and the prop howling 50 rpm above redline. From that initial data point, the test requires a reduction of engine speed in 50-rpm increments all the way down to 2000 rpm. Assuming care and precision of the pilot in finding the correct settings, this test can go quickly. A similar version of this test is performed at 10,000 feet msl.
Other tests include checking the take.off and initial climb at all normal flap settings, and checking the climb-power test all normally used engine speeds. (We did this one from 2400 to 2750 rpm.)
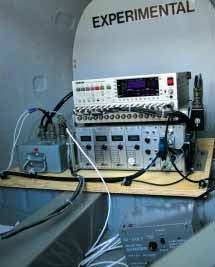
Finally, and likely the most enjoyable test, was what is called the gas-pressure test, described at the beginning of this story. Where most of the other tests have had the engine driving the propeller, the gas-pressure test ensures that the prop is driving the engine. This state can result in odd resonances at the higher, harmful frequencies. To recap: The test places the engine at idle, being driven by the prop, with a steep pitch down to obtain max rpm followed by a smooth but rapid pitch up to the stall; in this way, the vibration levels at a wide range of airspeeds can be sampled.
Crunching the Data
After every flight, one of the two Hartzell guys would sit down with the laptop and carefully scan the traces for big, ugly spikes. (The other would quickly start work preparing the test prop for the next test.) Generally, they want the peak vibration levels to be below a certain level. They know the actual values, of course, but they’d prefer that this information be kept in the family, as it were. Fortunately, the 74-inch prop showed no spikes in the “we’re concerned about that” region.
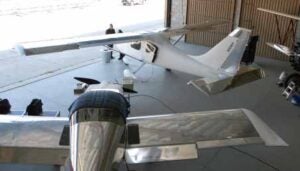
Thanks to the efficiency of the Hartzell system, and the guys’ good humor, we were able to test two props in four flying days. The 80-inch prop was found to have some very minor resonances in the gas-pressure test, and so will carry a manifold-pressure limitation with this engine. Naturally, at just the point of that resonance, the propeller/engine combo felt completely smooth. What’s more, there were often times during the testing that the engine seemed utterly content, and yet Lee would point out a wiggle on the real-time data indicating a rise above the baseline vibration. From the pilot’s seat, you’d never know.
After five days of testing and seeing the data, I’ve come down with a new sense of conservatism regarding metal props. And the reason is that the dynamics are just not evident from the cockpit. What may feel like a great combination could in fact be tearing itself to pieces.
For more information on Hartzell propellers, call 937-778-4379 or visit hartzellprop.com.