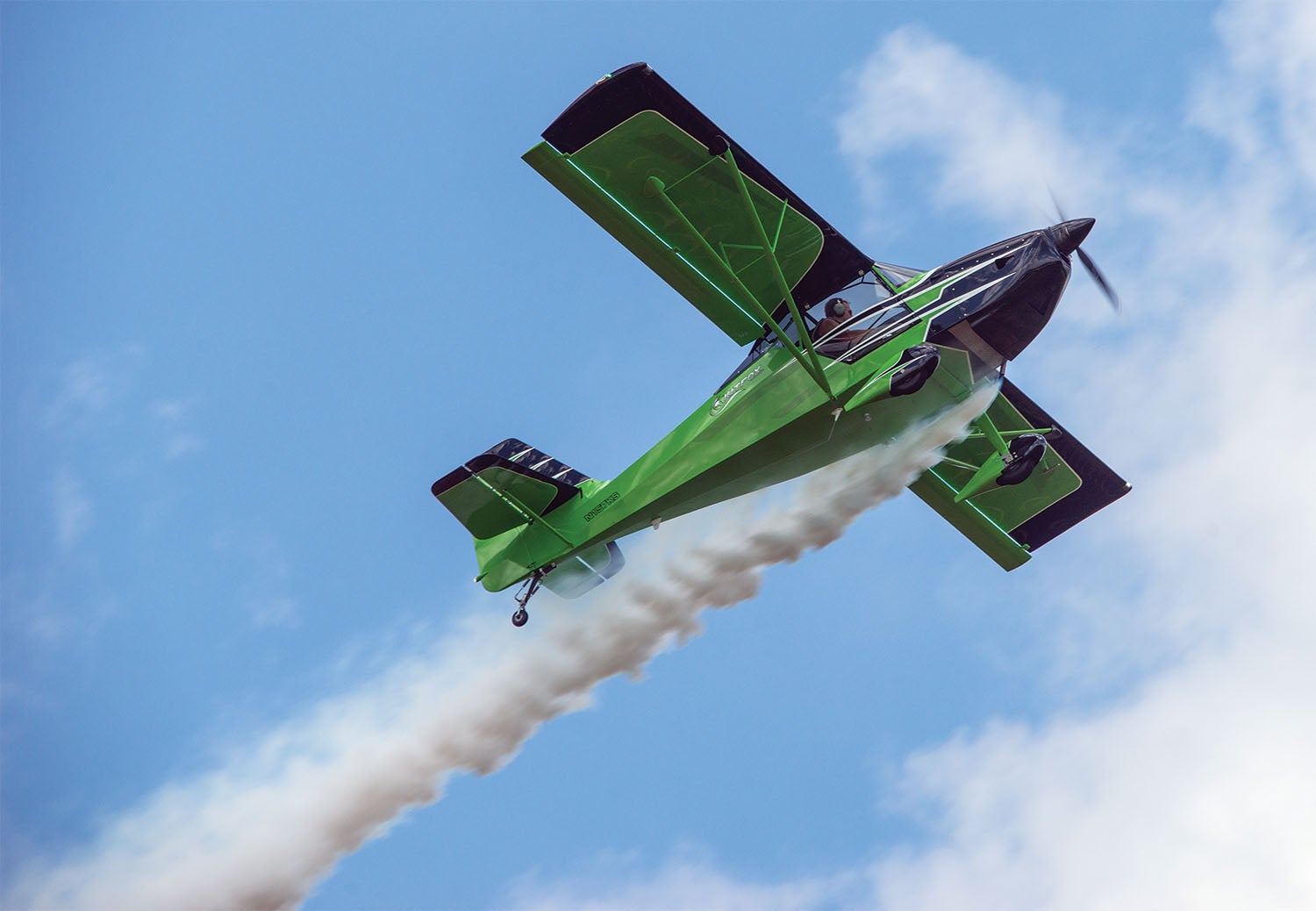
The horizontal tail has two functions. It provides control power to trim and maneuver the airplane in pitch, and it stabilizes the airplane about the longitudinal axis. This month we will take a look how the geometry of the horizontal tail affects its contribution to the stability of the airplane.
Horizontal Tail Effect on Stability
The horizontal tail is mounted to the aft end of the fuselage, well behind the center of gravity (CG) of the airplane. As the airplane pitches, its angle of attack changes. This change in angle of attack changes the lift of the tail. A nose-up pitch increases the angle of attack and hence the lift of the tail. This increase in lift pushes up on the aft end of the fuselage, producing a nose-down pitching moment that opposes the initial nose-up pitch.
A nose-down pitch produces the opposite effect. The lift of the tail decreases or becomes negative (depending on the angle of attack). This produces a nose-up pitching moment increment from the tail that drives the nose back up.
Geometric Effects
The stabilizing influence of the tail is a function of its geometry. There are several factors that affect how much the tail stabilizes the airplane.
Tail Arm
The first aspect of geometry that affects the stabilizing influence of the tail is the tail arm, which is the distance between the center of gravity and the aerodynamic center of the horizontal stabilizer. The tail arm determines how much leverage the tail has to generate pitching moment.
The longer the tail arm is, the more pitching moment a given increment of tail lift will provide. This holds true both for the stabilizing influence of the tail and the control power of the tail.
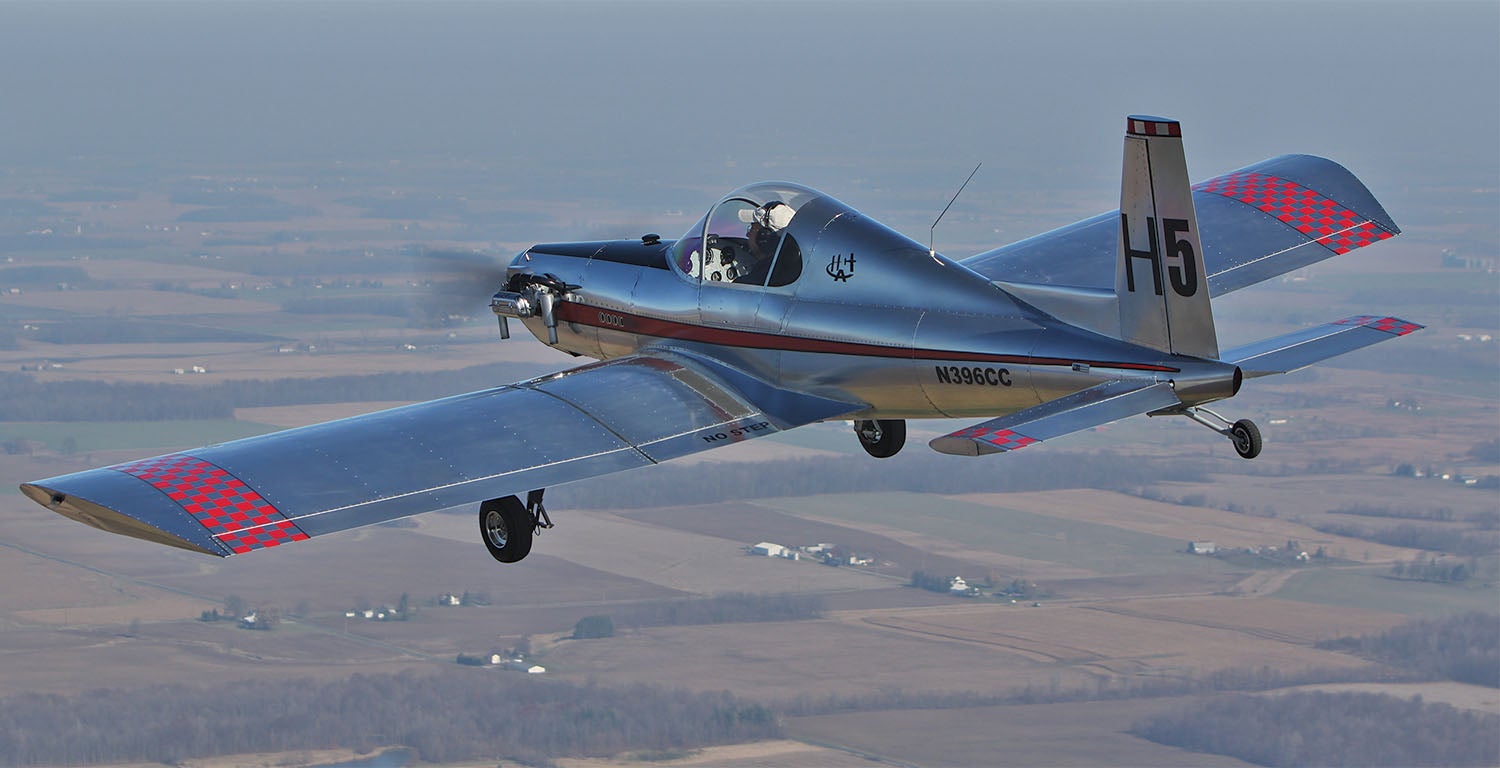
Tail Area
The second major parameter that affects the stabilizing influence of the tail is the area of the tail itself. The more area the tail has, the more lift increment it generates in response to a change in angle of attack and the greater its stabilizing influence.
Tail Volume Coefficient
The overall stabilizing effect of the horizontal tail is proportional to the area of the tail multiplied by the tail arm.
For design work, it’s useful to come up with a single parameter that can represent the stabilizing effect of the tail quantitatively. This parameter is a nondimensional number called the tail volume coefficient.
A nondimensional quantity has no units such as pounds, feet, etc. It is independent of the physical dimensions of the object or phenomenon it describes. The advantage of using nondimensional numbers is that it allows direct comparison and correlation between things of different size.
In much the same manner that lift and drag are represented by lift coefficient and drag coefficient, the tail volume coefficient captures the effect of the tail area, tail arm and wing geometry in a single parameter.
The stabilizing influence of the tail is proportional to the tail arm multiplied by the tail area. In dimensional terms, this number has dimensions of volume (length cubed), so it is referred to as “tail volume.”
We normalize this to get to a nondimensional coefficient by dividing by the product of the wing area times the main aerodynamic chord of the wing.
The resulting “tail volume coefficient” gives a measure of the stabilizing influence of the tail that is independent of the size of the airplane.
Vh = (St / Sw) * (Lt / MAC)
Vh = Horizontal tail volume coefficient
St = Horizontal tail area
Sw = Wing area
Lt = Tail arm
MAC = Wing mean aerodynamic chord
Tail volume coefficient is particularly useful because it takes into account not only the geometry of the tail, but the geometry of the wing. Both the relative area of the tail and the wing and the relative length of the wing MAC and the tail arm are significant in tail sizing.
The stability of the basic wing/body is a function of the position of the CG and the wing area. Usually the CG is not exactly at the aerodynamic center of the wing. If the CG is forward of the wing aerodynamic center, the lift acts behind the CG and the wing/body alone will be stable. If the CG is aft of the wing aerodynamic center, the influence of wing lift is destabilizing. The magnitude of the wing-induced stability increment is determined in large part by the wing area. The bigger the wing, the larger the lift (and pitching moment) increment from a change in angle of attack. The ability of the tail to stabilize the wing/body combination is accordingly a function of the relative size of the wing and tail as well as the tail arm.
Wing chord is also significant when sizing the horizontal tail. The constant (zero-lift) pitching moment generated by the wing is a function of the camber of the wing’s airfoil and is proportional to the MAC of the wing. The relative length of the MAC and the tail arm determines how much tail lift (or downforce) is needed to trim out the pitching moment generated by the wing.
The bigger the wing area and the longer the MAC, the more tail area and/or arm will be needed to properly stabilize and trim the airplane. The tail volume coefficient takes these effects into account directly.
Tail volume coefficient is commonly used for initial tail sizing. By working from simple drawings, it’s possible to determine the value of Vh for a new design by comparing it to existing airplanes of similar configuration. The top view of an airplane contains all of the information needed to make the calculation, and since Vh is nondimensional, the scale of the drawing does not matter.
Setting Vh to a target value that falls within the range set by historical data provides a good starting point for the new design. Later on, as the design becomes more defined, more detailed calculation of the CG range and stability characteristics of the new airplane will be used to refine the design of the tail.
Tail Aspect Ratio
The aspect ratio of the tail also affects stability. The stabilizing pitching moment generated by the tail is determined by how much the tail lift changes in response to a change in angle of attack.
The change in lift coefficient per degree of angle of attack is a function of aspect ratio. The higher the aspect ratio, the more the lift changes per degree of angle of attack change. This means that for a given tail area, a higher aspect ratio tail will provide a larger stability increment because its lift will vary more rapidly with angle of attack than a lower aspect ratio surface.
It’s important to note that, to the first order, the maximum lift the tail can generate does not change within the range of aspect ratio typically used for tail surfaces. This means that the maximum control power and trimming increment available from the tail is primarily a function of tail area, not tail aspect ratio.
A higher aspect ratio horizontal tail will make the airplane more stable in pitch than a lower aspect ratio surface. This does come at the cost of increased structural weight.
If stability is what is sizing the tail (typically the aft CG limit), a higher tail aspect ratio will allow the tail to be a bit smaller, thus reducing wetted area and parasite drag. If the tail is sized by control power (typically the forward CG limit), increased aspect ratio does not make it possible to use a smaller tail, and the extra weight of the higher aspect ratio surface actually makes the airplane heavier without providing any drag advantage.
It’s typical for the horizontal tail to have a somewhat lower aspect ratio than the wing. This is usually due to two factors. The first is that tails tend to be relatively thin, so a lower aspect ratio saves significant weight in the tail structure.
The second is that because the change in lift coefficient with angle of attack is lower for lower aspect ratio surfaces, they tend to stall at higher angles of attack than those of higher aspect ratio. Since stalling the tail at any angle of attack inside the normal flight envelop can lead to loss of control it’s important to ensure that the wing will always stall before the tail. Stall angle of attack is a function of many things including airfoil geometry, but using a tail with a lower aspect ratio than the wing helps ensure that the wing stalls first.
Thank you for the article!
The formula would better be:
Vh = (St/Sw)/(Lt / MAC)
Sorry,
Errata for my upper statement:
Vh = (St * Lt ) / (Sw * MAC)
Thanks José. We’ve corrected the expression in the article.